The Most Effective Way to Demonstrating RF Active Implant Safety
Problem Description

Undesirable interactions of an active implanted medical device (AIMD) inside an MRI scanner.
Magnetic resonance imaging (MRI) is a medical imaging modality, which is indispensable in diagnosing several pathologies. Nevertheless, the presence of medical implants in some patients taking an MRI scan may lead to undesirable interactions of the implants with the radiofrequency (RF) radiation necessary for the operation of the scanner. Therefore, it is necessary to develop a comprehensive risk assessment methodology, in order to determine the specific conditions that would permit an MRI examination for implant-bearing patients.
Standards Addressed
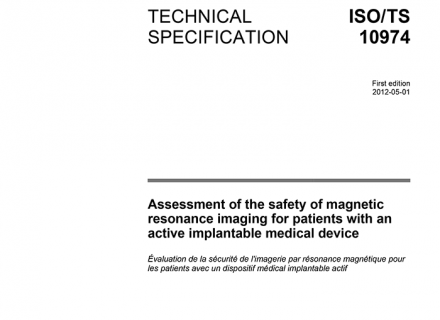
The standard ISO/TS 10974 for assessment of the safety of magnetic resonance imaging for patients with an active implantable medical device.
The ISO Technical Specification 10974 (ISO/TS 10974) defines the procedures to assess the local power deposition (RF heating) at the electrodes and the voltage/current (EMC) at the device terminals of an active implanted medical device (AIMD). Vertical standards define the risk assessment procedures. Members of ZMT and the IT’IS Foundation have contributed in the development of the standards and have optimized a toolbox for demonstrating RF implant safety.
IMAnalytics and MRIxViP are qualified by the FDA for MRI safety evaluations.
Methodology
1. Generation of the Calibrated AIMD Response Model (piX and MITS)
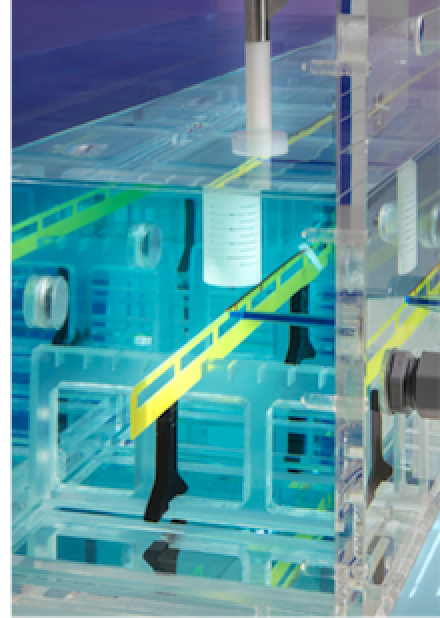
The piX system is used to validate numerical results for the transfer function.
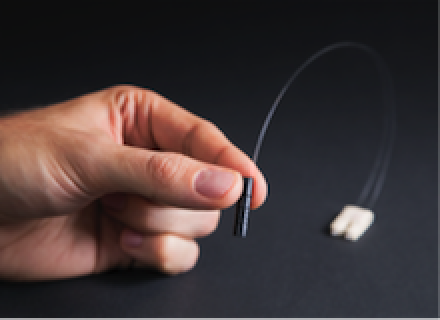
RFoF1P4MED: A miniature electrically fully isolated RF-over-Fiber (RFoF) transducer.
In the Tier 3 approach described in TS 10974 the first step towards safety evaluation is to create a response model of the AIMD, namely to create a transfer function that allows the assessment of the power deposited at the distal end of the AIMD lead or the current induced at the device terminals under known excitation.
The AIMD model can be determined either experimentally and/or with simulations. In the experimental case, a physical sample of the device under test (DUT) is measured with the piX system equipped with E1TDSz probe (RF Heating) or RFoF1P4MED probe (EMC). The photonic technology avoids crosstalk of the excitor with the DUT (E1TDSz probe) and allows measurement of voltages without modifying the devices (RFoF1P4MED). In numerical evaluation, Sim4Life electromagnetics solver and a CAD model of the DUT are used to simulate the AIMD model. The model is then calibrated to a well-defined exposure condition with a medical implant test system (MITS).
2. Validation of the AIMD Response Model for Power Deposition and for Induced Voltages or Current at the Device Terminals
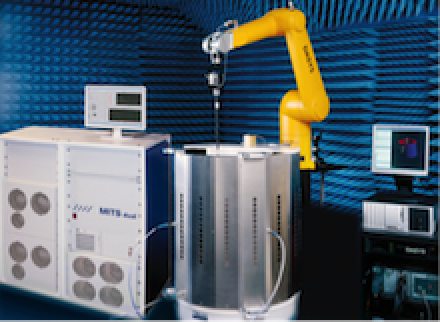
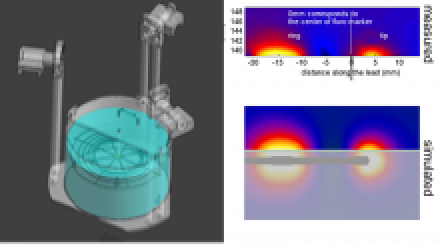
The MITS 1.5/3.0 validates the power deposition distribution along a pacemaker lead.
Thereafter, the created model needs to be validated with a sufficient set of orthogonal test functions. This involves specific absorption (SAR) or temperature rise (ΔΤ) measurements in RF heating case, or measurements of the current at the terminals and/or the induced voltages inside the device for EMC with RFoF1P4MED. The MITS allows for the fast evaluation of the AIMD model under worst-case incident fields of commercial scanners, but also for specific exposures generated by varying the polarization of the B1-field which is monitored continuously with TDS B1 measurements system.
The integration of the measurement and Sim4Life results is seamless for the user, facilitating comparison and sensitivity and/or uncertainty analysis. It should be noted here that the AIMD model can be established for each operating mode of the AIMD separately.
3. Computation of the Incident Field Distributions for the Patient Population
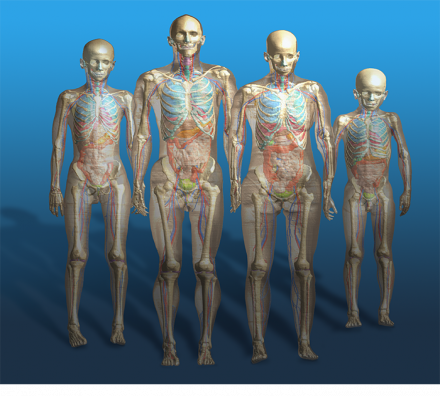
ViP3.0 human models.
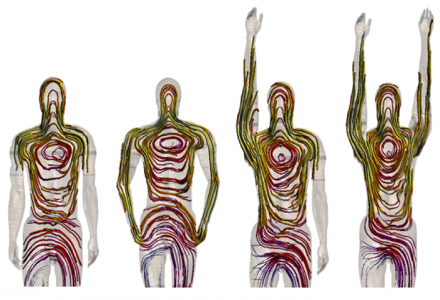
POSER tool in action.

Coil library includes E-fields of coils with varying length and diameter.
Once the AIMD response model is known and validated, the next step is to estimate typical incident field distributions with which the AIMD can be excited when implanted inside a human body (in vivo). The approach described in TS 10974 requires that the risk assessment of RF heating covers a wide range of the population.
Currently, Sim4Life is the only software platform that is based on computable anatomical phantoms functionalized for morphing and posing, enabling the user to obtain a wide and representative patient population with realistic and clinically relevant postures. The choice is not limited to standard male and female subjects in child- or adulthood, but includes an elderly male and an obese one, the latter being most important for worst-case evaluations inside an MRI volume coil.
The calculation of the incident electric fields for all the above computational models and for all the possible clinical exposure scenarios would be a challenging task in terms of human and computational resources. Instead of performing the calculations anew, the user has the option of acquiring MRIxViP, a validated library of the electric field distributions in eight human models placed at different imaging positions (according to TS 10974) inside various birdcage coils, which are carefully selected to represent the majority of commercially available ones.
IMAnalytics and MRIxViP are qualified by the FDA for MRI safety evaluations.
4. Power Deposition and Risk Assessment for RF Heating

IMAnalytics Module: automated Tier 3 analysis of implants (PiX transfer functions) with user-defined parameter ranges (e.g., normalizations, coil dimensions, shimming angles, clinical routings, subsets of the patient population and landmarks).

Tier 3 deposited power for different imaging positions.
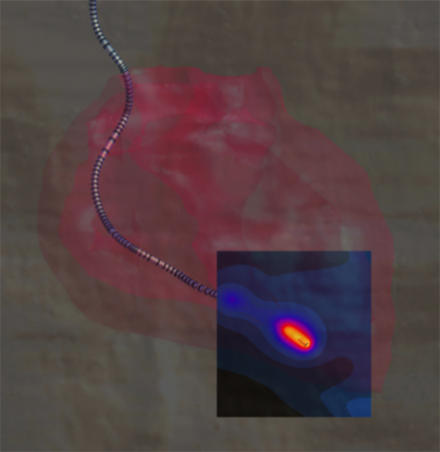
Temperature rise at the tip of a cardiac pacemaker lead, simulated with Sim4Life.
The incident field distributions are used to assess power deposition at the distal tip of the AIMD lead when implanted inside the human body.
The safety analysis as described in Tier 3 of TS 10974 requires repeating the power deposition assessment for a wide range of computational models, for all the possible ways of clinical implantation in them, and for all possible exposure scenarios. Performed manually, this would be a very demanding task in terms of human and computational resources.
With the IMAnalytics module of Sim4Life, this becomes a simple, reliable and traceable procedure. From the AIMD response model, the incident field distributions and the implants’ routing trajectories, IMAnalytics automatically performs the statistical analysis of power deposition at the tip of the AIMD lead in all possible scenarios. The results can be exported and included in regulatory submission reports.
The industry mainly applies two approaches to translate the power deposition into risk assessment. The first one is to use animal experiments by injecting the equivalent power to the electrodes and assessing the response (e.g., change in the pacing threshold) as a function of the deposited power. The other approach is to translate the power deposition into in vivo temperature rise inside the human tissues using the Sim4Life thermal solver, which has been validated both for local and locoregional RF heating in humans.
IMAnalytics and MRIxViP are qualified by the FDA for MRI safety evaluations.
Procedure Overview

Publications
- Zastrow, E., Cabot, E., Kuster, N. Assessment of local RF-induced heating of AIMDs during MR exposure (2014) 2014 31th URSI General Assembly and Scientific Symposium, URSI GASS 2014, art. no. 6930111.
- Cabot, E., Lloyd, T., Christ, A., Kainz, W., Douglas, M., Stenzel, G., Wedan, S., Kuster, N. Evaluation of the RF heating of a generic deep brain stimulator exposed in 1.5T magnetic resonance scanners (2013) Bioelectromagnetics, 34 (2), pp. 104-113.
- Kyriakou, A., Christ, A., Neufeld, E., Kuster, N. Local tissue temperature increase of a generic implant compared to the basic restrictions defined in safety guidelines (2012) Bioelectromagnetics, 33 (5), pp. 366-374.
- Neufeld, E., Kühn, S., Szekely, G., Kuster, N. Measurement, simulation and uncertainty assessment of implant heating during MRI (2009) Physics in Medicine and Biology, 54 (13), pp. 4151-4169.
- Gosselin, M.-C., Neufeld, E., Moser, H., Huber, E., Farcito, S., Gerber, L., Jedensjo, M., Hilber, I., Gennaro, F.D., Lloyd, B., Cherubini, E., Szczerba, D., Kainz, W., Kuster, N. Development of a new generation of high-resolution anatomical models for medical device evaluation: The Virtual Population 3.0 (2014) Physics in Medicine and Biology, 59 (18), pp. 5287-5303.